Section 15.1 Examples of Gyroscopic Phenomena
Engineers take advantage of gyroscopic effects in many industrial applications, such as in guidance and navigation systems of ships, airplanes and spacecraft or in motion stabilizers. Gyroscopic effects can also be detrimental and even dangerous to the proper behavior of vehicles or machinery.
Example 1: Rotary Engines on Airplanes. Rotary engines were early types of internal combustion engines, in which the crankshaft remained stationary in operation, with the entire crankcase and its cylinders rotating around it as a unit. Rotary engines were mounted on WWI biplane fighter aircrafts such as the Sopwith Camel. This large rotating mass behaved like a gyroscope and was the cause of stability and control problems in aircraft. Due to the direction of the engine’s rotation, left turns required effort and happened relatively slowly, combined with a tendency to nose up, while right turns were almost instantaneous, with a tendency for the nose to drop. The Sopwith Camel behavior caused inexperienced pilots to crash or stall during takeoff. See Figure 15.1.1.
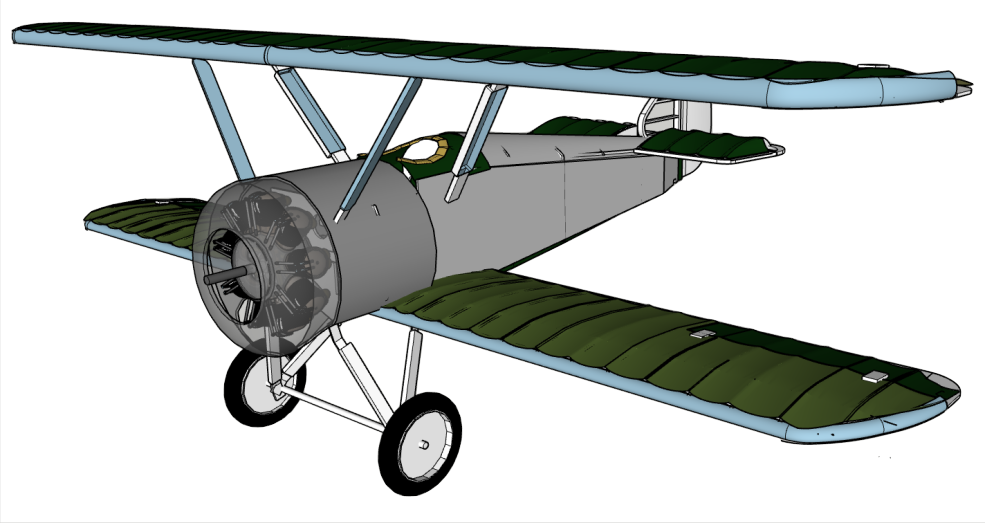
Example 2: Gyroscopic Effect of Propeller on Light Aircraft. The propeller of a single-engine light aircraft acts as a gyroscope: during a turn, the propeller causes the plane to tilt about the pitch axis. In a right turn and for an anti-clockwise rotation of the propeller (viewed from the pilot), the plane will have a tendency to rise. See Figure Figure 15.1.2.
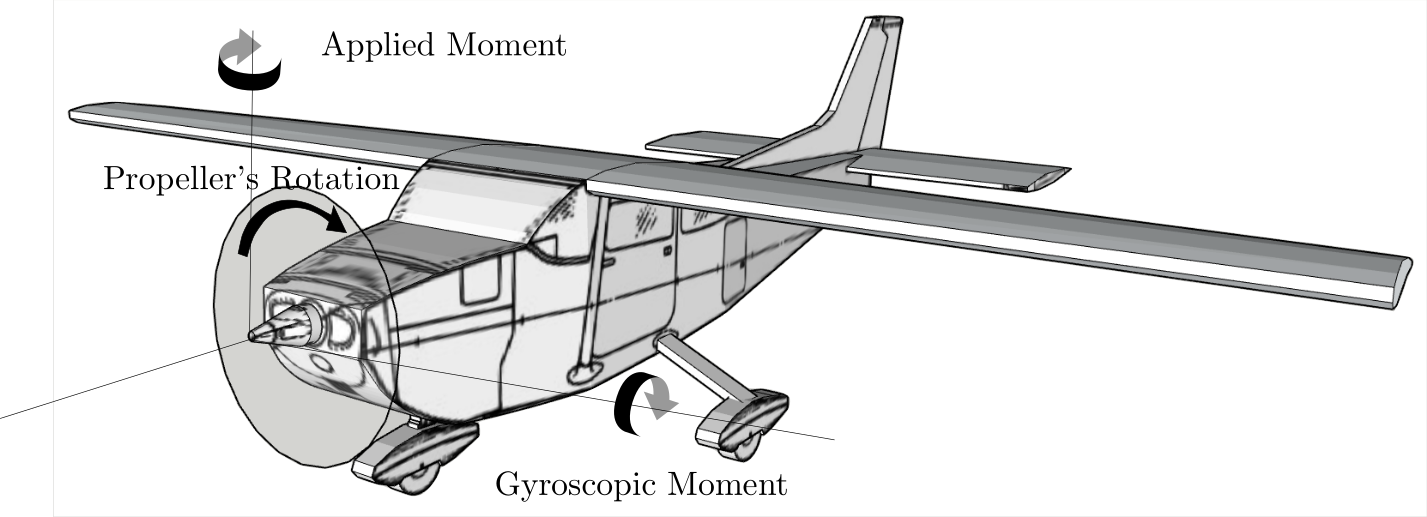
Example 3: Stability and Countersteering of Motorcycles. Above 20 km/h, the wheels of a motocycle traveling along a straight path endow the vehicle great stability: the rider can do all kinds of tricks without destabilizing the motocycle. Above 35 km/h, the axes of the wheels become so stiff, that it becomes impossible to turn the handlebar. Countersteering must be used by the rider to initiate a turn toward a given direction by momentarily steering counter to the desired direction. To negotiate a turn successfully, the combined center of mass of the rider and motorcycle must first be leaned in the direction of the turn, and steering briefly in the opposite direction causes that lean. The slight pressure applied by the rider on the right side of the handlebar leads to the leaning of the front wheel on his right side.
Example 4: Anti-Rolling Gyros. Ship stabilizing gyroscopes are a technology developed in the 19th century and early 20th century and used to stabilize roll motions in ocean-going ships such as the ocean liner SS Conte di Savoia, or the USS Henderson. Since the 1990s, interest in this technology has reemerged for low speed roll stabilization of vessels.
Example 5: Gyrocompasses. Gyrocompasses are navigation tools. Since gyroscopes maintain their direction, if they are mounted into a device that allows them to move freely, the gyroscope will still point in the same direction, irrespective of the motion of the body it is mounted on. The angular positions of the gyroscope can then be measured: it then acts like a compass. Gyrocompasses are commonly used in ships and aircraft. They can also be used as artificial horizon gauges to indicate the position of an aircraft relative to the horizon, that is, the pitch of the aircraft.
Example 6: Attitude Control of Satellites with Gyrodynes. These actuators are called gyrodynes or Control Moment Gyros. They exert a gyroscopic couple about mobile axes. Their use is very complex. A minimum of two gyrodynes is needed to achieve a control moment about a fixed axis. For arbitrary attitude control in 3D, an architecture of four gyrodynes with concurrent axes is needed. Such systems have a propensity to reach singular configurations where they lose their capacity. Special control laws must be devised to show that any configuration can be reached.
Other uses. Gyroscopes can be used to provide stability for the Segway. In Virtual Reality, gyroscopes are used in headsets and other VR products. Gyroscopes are used to keep complex robots upright. They are also present in smart phones, and hand-held video camera.